Laser-produced plasmas in magnetized ambients
Laser irradiation of a solid target embedded in a magnetized background plasma creates a dense plume moving at super-Alfvénic speeds though the ambient. Magnetic pulses launched by this "piston" are modified by the electromagnetic interactions of the debris plasma with the background plasma and can evolve into collisionless shock waves (Niemann, 2013; Schaeffer, 2012).
Collisionless Shocks
One of our main ongoing projects is the study of magnetized collisionless shocks. The activation of our three high-energy lasers- first PHOENIX in 2007, RAPTOR in 2010, and later PEENING in 2014- lead to a unique capability to launch large scale studies of space phenomena, such as collisionless shocks and related aspects of their physics.
Starting with 1960s when the Earth's bow shocks were first observed, the continuous interest in collision-free shocks and related phenomena is justified also by the abundance of their occurrences throughout space and astrophysical environments in a variety of situations: planetary bow shocks, supernova remnants, rotative magnetized plasmas near active galactic nuclei and pulsars, etc. Of great importance is also understanding their impact on the ambient medium through plasma heating, or cosmic ray generation, or space weather, as in the local cosmos due to solar hydromagnetic activity. In the process of particle acceleration to cosmic ray levels, energies above 100 MeV and reaching even GeV ranges (for solar energetic particles) can develop. It has been a grand challenge to create magnetized collisionless shocks of cosmic relevance in a laboratory due to a number of competing criteria that must be fulfilled. The relevance to the collision-free interactions in space is achieved in laboratory experiments only by a proper scaling of the relevant parameters. The table below explains how we can follow the same laws of physics, even though the length of our experiments, velocities, densities or magnetic fields cannot be matched to the cosmic scales. Of critical importance are the dimensionless parameters.
Scaling of relevant parameters in laboratory experiments to Earth's bow shock conditions:
Plasma Parameter | Earth's Bow Shock | Laboratory Experiment |
---|---|---|
Initial Debris Expansion Speed | 400 km/s | 400 km/s |
System Size | 100-1000 km | 50 cm |
Ambient Ion Density | 5 cm-3 | 5 x 1013 cm-3 |
Magnetic Field, B0 | 5 x 10-5 Gauss | 500 Gauss |
Ambient Ion Inertial Length | 100 km | 3 cm |
Debris Ion Directed Gyroradius | 835 km | 12 cm |
Dimensionless Plasma Parameter | Earth's Bow Shock | Laboratory Experiment |
Alfvénic Mach Number | 8 | 4 |
Magnetic Reynolds Number | 105 | 103 |
Debris-Ambient Coupling | 1.2 | 2 |
Magnetic field generation via Biermann battery mechanism
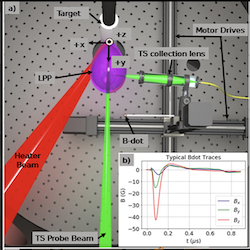
The evolution of magnetic fields from formation to amplification and saturation is an important problem in plasma physics (including high-energy density plasmas, inertial confinement fusion research, etc) and astrophysics. The cosmological magnetogenesis is since long an outstanding question due to the important role that magnetic fields play in the cosmic dynamics, and also its presence in much of the observable universe.
Magnetization of cosmic space is complex and various processes are likely to contribute to it- L. Biermann in 1950 for instance proposed a triple-phase process for the field generation and amplification, as well as Kulsrud later in 1997.
A favored scenario for the early universe magnetization is the seeding of fields by a Biermann mechanism at initial 10-20 Gauss, and further amplification to micro-Gauss by other mechanisms, such as turbulent dynamo or even more likely Weibel instabilities.
The high-repetition rate capability of one of our lasers (Peening laser) enables us to study in detail the formation of Biermann fields over large scales (Pilgram, 2022). We have extended the work of prior experiments, which spanned over millimeter scales, by spatially measuring magnetic fields in multiple planes on centimeter scales over thousands of laser shots. The image on the right shows a top view of our experiment, with the driver Peening beam in red, a probe laser beam for plasma diagnostics (Thomson scattering) in green, and the plasma plume expansion in purple. The magnetic flux probe is moved automatically in synch with the laser beams (1 Hz) using the motorized translating stages.
Measurements with magnetic flux (B-dot) probes show azimuthally symmetric magnetic fields that range from 60 G close to the target to 7 G at 4.2 cm from the target. The expansion rate of the magnetic fields and evolution of current density structures are also examined. Correlations between electron density and temperature, measured at different coordinates in the plasma via a raster Thomson scattering diagnostic, with the probed magnetic field give more insights into the field evolution.
Plasma Diagnostics
- Thomson scattering in high- and low-density plasmas for temperature and density measurements
- Laser-induced fluorescence for ion velocity distribution measurements in laser plasmas
- Schlieren imaging of shock waves in gas backgrounds
- Magnetic field measurements using B-dot probes, Faraday rotation, and other diagnostics
Points of interest in our research projects:
- Characterization of diamagnetic cavities
- Turbulent and laminar coupling to the ambient
- Langmuir turbulence at the "piston" boundaries
- Volumetric reconstruction of magnetic and electric dynamics
- Wave instabilities in configurations parallel to the magnetic field
- Parallel collisionless shocks formation and structure
- Validation of hybrid 2D and 3D numerical simulations of collisionless interactions
- Energy transport in magnetized plasmas